The quest for sustainable and renewable energy sources has intensified in recent years, with solar energy emerging as a front-runner in this global transition. At the heart of solar energy technology are photovoltaic (PV) systems, a sophisticated yet elegantly simple method for harnessing the sun’s energy. This section delves into the basics of solar energy and the role of photovoltaic technology in converting this abundant natural resource into usable electricity.
The Essence of Solar Energy
Solar energy is the light and heat radiated from the Sun, a colossal fusion reactor that has been burning for over 4.5 billion years. It offers a clean, inexhaustible, and increasingly cost-effective source of power. Solar energy is key in addressing the pressing environmental challenges of our time, such as climate change and fossil fuel depletion.
Introduction to Photovoltaic Technology
Photovoltaic technology, at its core, is the process of converting sunlight into electricity. The term ‘photovoltaic’ combines two words: ‘photo,’ meaning light, and ‘voltaic,’ pertaining to electricity. This technology is built upon the photovoltaic effect, a phenomenon discovered in the 19th century, where certain materials generate an electric current when exposed to sunlight.
Key Components of Solar Panels
A typical solar panel consists of multiple solar cells, which are the fundamental units that convert sunlight into electrical energy. These cells are often made from semiconductor materials, such as silicon, which plays a critical role in the photovoltaic process. Encased in protective materials, these cells are designed to withstand environmental factors while efficiently capturing solar energy.
The Significance of Photovoltaic Technology
Photovoltaic technology has revolutionized the way we harness and utilize solar energy. It’s a cornerstone of renewable energy strategies, offering a path towards reducing greenhouse gas emissions and dependence on non-renewable resources. The adaptability of PV systems, from small-scale rooftop installations to large solar farms, underscores their importance in the global energy landscape.
The Evolution of Solar Panels
Solar panel technology has evolved significantly since its inception. Early solar cells, while groundbreaking, were limited in efficiency and practicality. Today, advances in materials science and engineering have led to more efficient, durable, and cost-effective solar panels. This continuous improvement is vital for meeting the growing global energy demand sustainably.
Understanding the Structure of Solar Panels
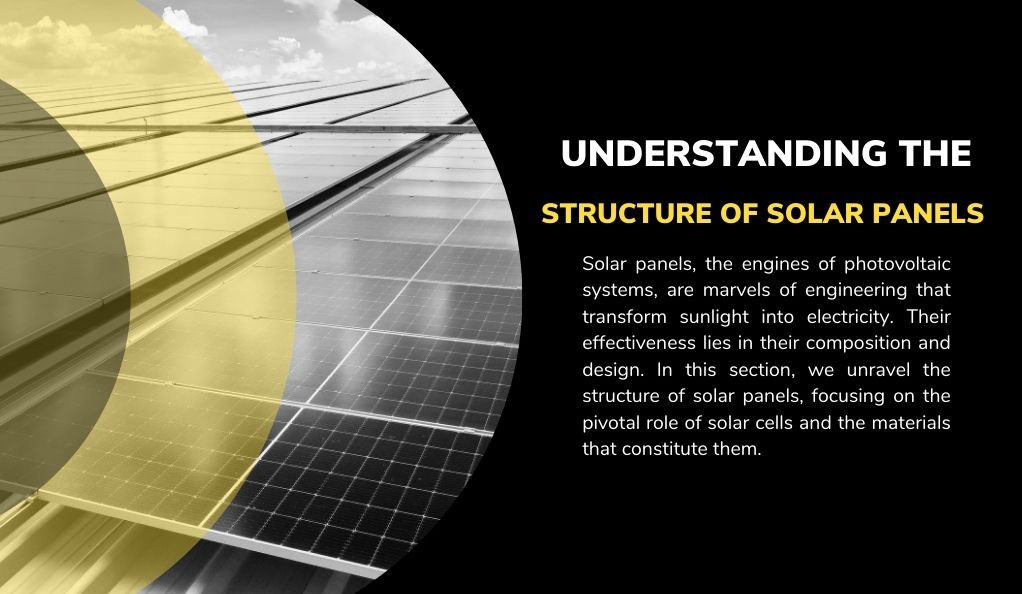
Solar panels, the engines of photovoltaic systems, are marvels of engineering that transform sunlight into electricity. Their effectiveness lies in their composition and design. In this section, we unravel the structure of solar panels, focusing on the pivotal role of solar cells and the materials that constitute them.
The Composition of Solar Panels
Solar panels are essentially assemblies of numerous solar cells linked together. Each solar cell is a thin slice of semiconductor, typically silicon, meticulously crafted to convert sunlight into electricity.
Solar Cells: The basic building blocks of a solar panel, these cells are where the magic of the photovoltaic effect takes place. Each cell generates a small amount of electricity, and when combined, they produce a significant amount of power.
Semiconductor Material: Silicon is the most widely used material in solar cells due to its abundance and photovoltaic efficiency. The cells consist of a positively charged layer and a negatively charged layer, which together create an electric field.
Silicon: The Semiconductor of Choice
Silicon’s atomic structure makes it ideal for solar cells. Each silicon atom has four valence electrons, which form stable bonds with neighboring atoms, creating a crystal lattice. This structure is essential for the photovoltaic process.
- Crystalline Silicon: Used in most solar cells, crystalline silicon can be either monocrystalline or polycrystalline. Monocrystalline silicon, with a uniform crystalline structure, offers higher efficiency but at a higher cost. Polycrystalline silicon, made from multiple silicon crystals, is less efficient but more affordable.
- Silicon Purity: The efficiency of a solar cell is directly related to the purity of the silicon used. Higher purity allows for better electron mobility, thereby enhancing the cell’s efficiency.
Design and Efficiency
The design of solar panels is aimed at maximizing efficiency and durability:
- Protective Layering: Solar cells are sandwiched between protective materials like glass and durable plastics to shield them from environmental elements while allowing sunlight to penetrate efficiently.
- Anti-Reflective Coating: This coating on the cells ensures that sunlight is absorbed rather than reflected, thereby increasing the panel’s efficiency.
- Efficiency Factors: The efficiency of solar panels is influenced by various factors, including the type of silicon used, the quality of manufacturing, and the presence of any impurities in the cells.
The Photovoltaic Effect: Core of Solar Energy Conversion
At the heart of a solar panel’s ability to convert sunlight into electrical energy is the photovoltaic effect. This remarkable process is the cornerstone of solar energy technology and is crucial in understanding how solar panels function. Let’s delve into the details of the photovoltaic effect and its vital role in solar energy conversion.
What is the Photovoltaic Effect?
The photovoltaic effect is a physical and chemical phenomenon where certain materials generate electric current or voltage upon exposure to light. This effect is the basic operational mechanism of photovoltaic cells, the building blocks of solar panels.
The Role of Semiconductor Materials
In solar cell technology, a semiconductor is a material exhibiting electrical conductivity levels between conductors and insulators. Silicon, a widely used semiconductor, plays a pivotal role in solar cells. Semiconductors possess energy bands, specifically the valence band and the conduction band. The photovoltaic effect, central to solar energy conversion, entails the transition of electrons from the valence band to the conduction band within these semiconductors.
Photovoltaic Process: Step-by-Step
- Absorption of Light: When sunlight strikes a solar cell, it is absorbed by the semiconductor material.
- Excitation of Electrons: The energy from the absorbed light excites electrons, enabling them to jump from the valence band to the conduction band.
- Creation of Electron-Hole Pairs: This excitation creates an electron-hole pair. The electron becomes a free carrier, able to move and carry an electrical current.
- Generation of Electric Current: An internal electric field in the solar cell causes these free electrons to move in a specific direction. This movement of electrons constitutes an electric current.
- Flow of Electricity: This current is then captured by conductors attached to the solar cell, leading to an external load where electricity can be utilized.
Efficiency and Influencing Factors
The efficiency of a solar cell in converting light into electricity depends on several factors:
- Material Properties: The type of semiconductor material and its purity greatly affect efficiency.
- Wavelength of Light: Not all light wavelengths are equally effective in producing the photovoltaic effect. The semiconductor’s properties determine which wavelengths are most efficiently converted.
- Temperature: The performance of solar cells can vary with temperature, usually decreasing with an increase in temperature.
The Role of Silicon and Doping in Solar Cells
Silicon’s pivotal role in the photovoltaic process cannot be overstated. This section explores why silicon is the preferred material for solar cells and how the process of doping enhances its efficiency in converting sunlight into electricity.
Why Silicon?
- Abundance and Accessibility: Silicon is the second most abundant element in the Earth’s crust. Its wide availability makes it an ideal candidate for widespread solar cell production.
- Energy Band Gap: Silicon has an energy band gap that is well-suited for photovoltaic applications. This band gap allows for efficient absorption of sunlight and the generation of a significant amount of electrical current.
- Stability and Durability: Silicon cells offer a robust and long-lasting solution, capable of withstanding various environmental conditions while maintaining efficiency over time.
The Doping Process
- What is Doping?: Doping involves introducing small amounts of other elements into the silicon to alter its electrical properties.
- Types of Doping:
- N-type Doping: Involves adding elements like phosphorus, which have more valence electrons than silicon. This results in excess free electrons, making the silicon layer negatively charged.
- P-type Doping: Involves incorporating elements such as boron, which have fewer valence electrons than silicon. This creates ‘holes’ or positive charge carriers in the silicon layer.
- Creation of P-N Junction: The combination of p-type and n-type layers forms a p-n junction. This junction is critical in creating an electric field, which is fundamental in the operation of a solar cell.
Electronegativity and Electron Flow
Electronegativity quantifies an atom’s capacity to attract and retain electrons. In silicon-based solar cells, differences in electronegativity between doped layers generate an electric field at the junction. When photons from light excite electrons, this electric field propels electrons towards the n-type layer and holes towards the p-type layer. This separation of charges establishes the conditions required for the generation of electric current, a fundamental process in solar energy conversion.
Efficiency Considerations
Efficiency in solar cells hinges on the precise distribution of electrons and holes, necessitating proper doping. Silicon, although effective, has efficiency limitations. Ongoing research seeks alternative materials and doping techniques to potentially achieve higher solar cell efficiencies and advance solar energy technology.
From Sunlight to Electricity: The Conversion Process
The ability of solar panels to convert sunlight into electricity is a marvel of modern engineering and physics. This section delves into the intricate processes that occur within a solar panel, transforming the energy of light into usable electrical power.
The Initial Interaction with Sunlight
The initial and crucial step in solar energy conversion involves the absorption of photons from sunlight by the photovoltaic cells. This absorption of photons is followed by the excitation of electrons within the semiconductor material, usually silicon. These electrons gain sufficient energy from the absorbed photons to break free from their atomic bonds, initiating the generation of electric current and the conversion of solar energy into usable electrical power.
Creation of Electron-Hole Pairs
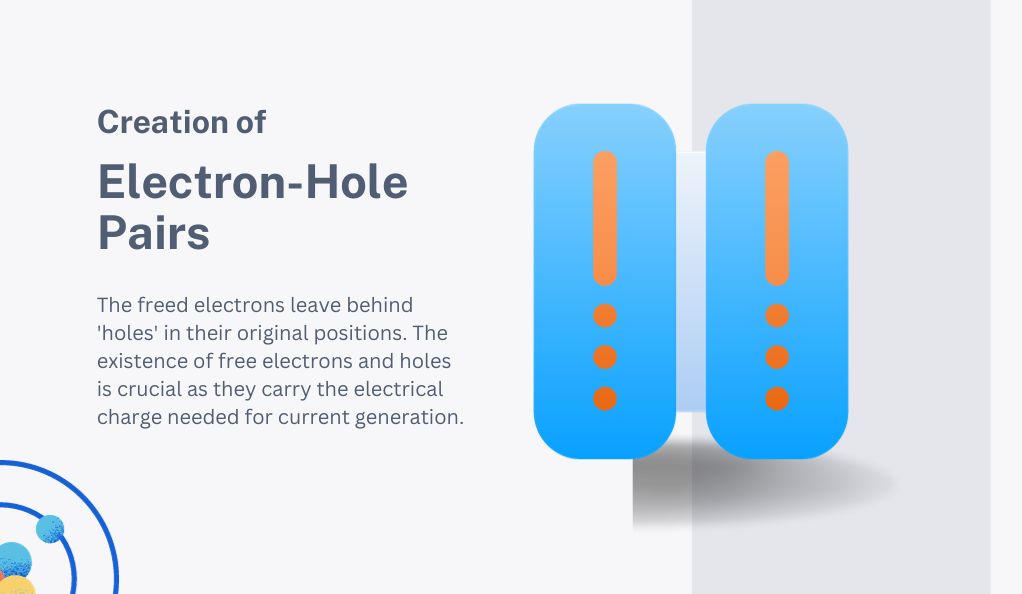
The freed electrons leave behind ‘holes’ in their original positions. The existence of free electrons and holes is crucial as they carry the electrical charge needed for current generation.
Formation of the Electric Field
- P-N Junction: The juxtaposition of p-type and n-type silicon layers creates a p-n junction. This junction is vital for establishing an electric field.
- Role of the Electric Field: This field acts as a directional force, pushing free electrons towards the n-type layer and holes towards the p-type layer.
Generation of Electric Current
As electrons migrate towards one layer and holes move towards another within the solar cell, they initiate an electrical current flow. This movement sets the stage for the conversion of solar energy into usable power. Subsequently, the generated electric current is directed out of the solar cell into an external circuit. There, it can either power electrical devices directly or be stored for future use, making solar cells a vital component of renewable energy systems.
Factors Affecting Conversion Efficiency
- Photon Wavelengths: Not all photons carry the same amount of energy. The ability of a solar cell to convert light into electricity depends on the wavelengths of light it can absorb.
- Thermal Losses: Some of the energy from the photons is lost as heat, which can affect the overall efficiency of the solar panel.
- Recombination of Electrons and Holes: Sometimes, electrons recombine with holes before they can contribute to the electric current, leading to efficiency losses.
Solar Panel Efficiency and Technological Developments
Solar panel efficiency is a measure of how well they convert sunlight into electricity. This section examines the factors influencing this efficiency and the technological advancements that continue to enhance it.
Understanding Solar Panel Efficiency
- Definition: Solar panel efficiency is the ratio of the energy output from the solar panel to the energy input from the sun. It is expressed as a percentage.
- Current Efficiency Ranges: The efficiency of commercial solar panels typically ranges from 15% to 22%. However, laboratory prototypes and advanced models have achieved efficiencies above 40%.
- Key Influencing Factors:
- Material Quality: The purity and type of the semiconductor material, usually silicon, play a significant role.
- Cell Design: The architecture of the solar cell, including its light absorption capabilities and electrical conductivity, affects efficiency.
- Surface Coating: Anti-reflective coatings and surface texturing help reduce light reflection and increase light absorption.
Advances in Photovoltaic Cell Technology
Solar cell technology can be categorized into three generations. First-generation solar cells, primarily silicon-based, like monocrystalline and polycrystalline, strike a balance between efficiency and cost, making them widely used. Second-generation, or thin-film solar cells, employ materials like cadmium telluride or amorphous silicon, offering cost-effectiveness and flexibility at the expense of efficiency. Third-generation cells leverage advanced materials such as perovskite, organic photovoltaics, or quantum dots, holding promise for higher efficiencies and potentially reduced production costs, driving innovation in solar energy technology.
Breakthroughs and Future Trends
- Perovskite Solar Cells: These have shown potential for high efficiency and low production costs. They could be used on their own or as a layer on top of traditional silicon cells to capture more light.
- Tandem Solar Cells: These cells combine multiple layers of different materials to capture a broader range of the solar spectrum, thereby increasing efficiency.
- Flexible and Transparent Solar Cells: Innovations in flexible and transparent cells could lead to their integration into windows, buildings, and even clothing.
- Concentrated Photovoltaic (CPV) Systems: These systems use lenses or mirrors to concentrate sunlight onto high-efficiency solar cells. They are more efficient but also more complex and costly.
Solar Panels in Use: Residential, Commercial, and Utility Applications
Solar panels have transcended their early niche status to become a mainstream energy source. This section highlights their diverse applications, from residential rooftops to large-scale utility projects.
Residential Solar Panels
- Rooftop Installations: The most common use of solar panels in residential settings is rooftop installations. These systems can either be grid-tied, allowing homeowners to sell excess electricity back to the grid, or off-grid, providing complete energy independence.
- Factors Influencing Installation:
- Roof Orientation and Space: Optimal solar panel installations require sufficient, properly oriented roof space.
- Energy Needs and Storage: System size is determined by household energy needs and the possibility of including battery storage for energy use at night or during cloudy days.
- Local Regulations and Incentives: Local building codes, zoning laws, and incentives can significantly impact the feasibility and economics of a residential solar installation.
Commercial Solar Applications
Businesses implement solar solutions in various ways, from on-site installations on rooftops or unused land, reducing operating costs and highlighting sustainability efforts, to participating in community solar projects when space is limited. Key considerations for businesses include assessing the return on investment (ROI), factoring in energy savings, maintenance expenses, and potential tax incentives. Additionally, embracing solar power aligns with corporate responsibility, enhancing a company’s reputation as an environmentally conscious entity, which can be advantageous in today’s market.
Utility-Scale Solar Farms
- Massive Energy Production: Utility-scale solar farms are large, ground-mounted installations that generate electricity on a massive scale, often to supply power to the grid.
- Key Features:
- Location: Typically located in areas with high solar irradiance.
- Size: These installations can cover hundreds of acres and require substantial investment and planning.
- Integration with the Grid: Utility solar farms are integrated into the existing power grid, providing a significant portion of the renewable energy supply.
Conclusion
In this comprehensive exploration of solar panel technology, we’ve unveiled the intricate processes behind their ability to convert sunlight into electricity. From the fundamental principles of the photovoltaic effect to the role of silicon and doping in enhancing efficiency, each aspect reveals the sophistication of this sustainable technology. We’ve also observed how solar panels adapt across various applications, from residential rooftops to large-scale utility projects. The continuous advancements in efficiency and design highlight solar panels’ growing significance in our journey towards a more sustainable and environmentally conscious future, making them an indispensable part of our renewable energy landscape.