Introduction to Solar Cell Efficiency
Solar energy has emerged as a powerful and sustainable source of electricity generation, paving the way for a greener and cleaner future. At the heart of this revolution lies the remarkable technology of solar cells, which convert sunlight into electrical energy. However, not all solar cells are created equal, and their efficiency plays a pivotal role in determining how much electricity can be generated from a given amount of sunlight.
The Significance of Solar Cell Efficiency
Solar cells, also known as photovoltaic cells, are the building blocks of solar panels. They are responsible for capturing photons from sunlight and transforming them into electrons, generating an electric current. The efficiency of a solar cell indicates how effectively it can convert sunlight into electricity. In essence, it quantifies the cell’s ability to harness the energy contained in sunlight.
Solar cell efficiency is a critical factor for several reasons:
- Maximizing Energy Output: A more efficient solar cell can generate more electricity from the same amount of sunlight. This means that a higher efficiency solar panel can produce more power in a given space, making it an attractive choice for residential, commercial, and industrial applications.
- Reducing Costs: Improved efficiency translates to greater energy production with fewer solar panels, reducing the overall cost of a solar power system. This not only benefits consumers but also contributes to the affordability of renewable energy solutions.
- Environmental Impact: Solar energy is one of the cleanest sources of electricity. Higher efficiency solar cells require fewer raw materials and occupy less space, resulting in a lower environmental footprint during manufacturing and installation.
- Grid Independence: Highly efficient solar cells enable off-grid applications in remote areas where traditional power sources are unavailable. They provide a reliable source of electricity for various purposes, from powering remote sensors to electrifying rural communities.
- Technological Advancements: The pursuit of greater efficiency drives innovation in solar cell technology. Research and development efforts are continuously focused on enhancing efficiency, leading to breakthroughs that benefit the entire renewable energy industry.
The Science Behind Solar Cells
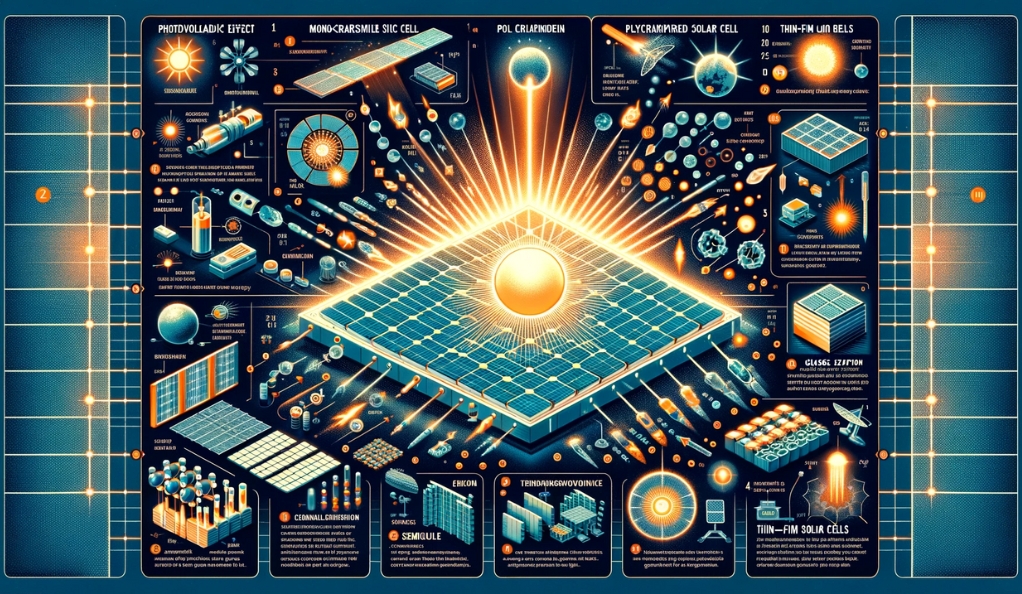
To truly understand solar cell efficiency, it’s essential to grasp the fundamental science behind how solar cells work. Solar cells are semiconductor devices that convert sunlight, composed of photons, into electrical energy. This conversion process is based on the photovoltaic effect, which was first observed by Alexandre-Edmond Becquerel in 1839 and has since become the cornerstone of solar technology.
How Solar Cells Generate Electricity
The operation of a solar cell can be broken down into several key steps:
- Photon Absorption: When sunlight reaches the solar cell, photons from the sunlight are absorbed by the semiconductor material within the cell. This absorption process excites electrons in the material, causing them to move to a higher energy state.
- Electron-Hole Pair Formation: The excited electrons leave behind “holes” or vacancies in the semiconductor’s atomic structure. This creates electron-hole pairs, which are essentially mobile charge carriers.
- Charge Separation: Due to the semiconductor’s internal electric field, the separated electrons and holes move in opposite directions. Electrons migrate toward the cell’s front surface, while holes move toward the back surface.
- Current Flow: The motion of electrons and holes generates an electric current, creating a flow of electricity within the solar cell.
- Electrical Output: The generated electricity is collected by metal contacts on the cell’s surface, which then feeds into external circuits for various applications.
Types of Solar Cells
Solar cells come in various types, each with its unique structure and materials. The most common types include:
- Monocrystalline Silicon Cells: These are made from a single crystal structure, offering high efficiency and long-term stability.
- Polycrystalline Silicon Cells: Created from multiple crystal structures, they are cost-effective but slightly less efficient than monocrystalline cells.
- Thin-Film Solar Cells: Thin-film cells use various materials like amorphous silicon, cadmium telluride, or copper indium gallium selenide (CIGS). They are lightweight and suitable for flexible applications.
- Multi-Junction Solar Cells: These advanced cells stack multiple layers of semiconductor materials to capture a broader spectrum of sunlight, resulting in higher efficiency.
Efficiency and Solar Cell Performance
Solar cell efficiency is directly related to how effectively the cell converts sunlight into electricity. It is expressed as a percentage and represents the ratio of electrical output to the total energy from absorbed sunlight. For example, if a solar cell has an efficiency of 20%, it converts 20% of the sunlight it receives into electricity.
The efficiency of a solar cell depends on several factors, including the quality of the semiconductor material, the cell’s design, and external conditions such as temperature and shading. Maximizing efficiency is a constant pursuit in the solar industry, as it directly impacts the cost-effectiveness and environmental benefits of solar power systems.
Measuring Solar Cell Efficiency
Now that we have a foundational understanding of how solar cells work, let’s delve into the methods used to measure their efficiency. Solar cell efficiency is a critical parameter because it provides valuable insights into the performance and quality of these energy-harvesting devices.
Parameters for Measuring Efficiency
Solar cell efficiency is determined by comparing the electrical output of the cell to the total energy from absorbed sunlight. To calculate efficiency accurately, several parameters are considered:
- Short-Circuit Current (Isc): This represents the maximum current a solar cell can deliver when its terminals are short-circuited, i.e., when the voltage across the cell is zero. Isc is measured in amperes (A).
- Open-Circuit Voltage (Voc): Voc is the maximum voltage across a solar cell when no current is flowing through it, i.e., when its terminals are open. Voc is measured in volts (V).
- Fill Factor (FF): The fill factor is a dimensionless parameter that quantifies how effectively a solar cell converts sunlight into electricity. It is calculated as the ratio of the maximum power the cell can generate (at its maximum power point) to the product of Voc and Isc.
- Maximum Power Point (Pmax): Pmax is the maximum electrical power output of the solar cell, usually achieved at a specific combination of voltage and current.
- Solar Spectrum and Light Intensity: The efficiency measurement should account for the solar spectrum and light intensity under which the cell is tested. Standard test conditions (STC) are often used, which include a spectrum similar to sunlight and an intensity of 1000 watts per square meter (W/m²).
Efficiency Calculation Formula
Solar cell efficiency (η) is calculated using the following formula:
η = (Pmax / (Isc * Voc)) * 100%
Where:
- η is the solar cell efficiency (expressed as a percentage).
- Pmax is the maximum power point (in watts) achieved by the cell.
- Isc is the short-circuit current (in amperes).
- Voc is the open-circuit voltage (in volts).
Significance of Efficiency Measurements
Efficiency measurements are crucial for several reasons:
- Quality Assurance: Manufacturers use efficiency measurements to ensure the quality and performance of their solar cells. High-efficiency cells are more desirable in the market.
- Comparative Analysis: Efficiency values allow consumers and businesses to compare different solar cell models and choose the most suitable ones for their needs.
- Performance Monitoring: Solar power systems use efficiency data to monitor the performance of individual solar panels and the overall system. Any decrease in efficiency can indicate issues that need attention.
- Research and Development: Efficiency measurements drive research and development efforts in the solar industry. Scientists and engineers strive to improve efficiency through technological advancements.
- Government Incentives: In some regions, government incentives and subsidies are based on the efficiency of solar panels. Higher efficiency panels may qualify for more significant incentives.
Factors Affecting Solar Cell Efficiency
Solar cell efficiency is not a fixed value and can vary under different conditions. Several factors can influence the performance of solar cells, either positively or negatively. Understanding these factors is crucial for optimizing the efficiency of solar power systems.
1. Temperature
Solar cell efficiency decreases as temperature rises. This phenomenon is due to the intrinsic properties of semiconductor materials. Higher temperatures lead to increased electron-hole recombination rates, reducing the cell’s overall efficiency. It’s essential to consider this effect when designing solar installations, especially in regions with hot climates.
2. Shading
Shading is a significant efficiency deterrent. Even partial shading of a solar cell can lead to a significant drop in output. When a portion of a cell is shaded, it operates in a reduced voltage state, causing other cells in the same module to work harder to compensate. To mitigate shading issues, solar panels are often designed with bypass diodes that allow current to flow around shaded cells.
3. Dust and Dirt
The accumulation of dust, dirt, or other debris on the surface of solar panels can reduce efficiency. These obstructions block sunlight from reaching the cells, diminishing their ability to generate electricity. Regular cleaning and maintenance are essential to ensure optimal performance.
4. Angle and Orientation
The angle at which solar panels are installed and their orientation relative to the sun also affect efficiency. To capture the maximum amount of sunlight throughout the day, panels should be tilted at an angle and oriented to face the sun’s path. Tracking systems that adjust panel angles throughout the day can further optimize energy capture.
5. Material Quality
The quality of the semiconductor material used in solar cells is critical. High-quality materials exhibit fewer defects and offer better electron mobility, resulting in higher efficiency. Monocrystalline and multi-junction solar cells are known for their material quality and efficiency.
6. Spectral Matching
Solar cells are most efficient at capturing specific wavelengths of light. The solar spectrum changes throughout the day, with variations in the distribution of sunlight. Spectral matching ensures that solar cells are designed to capture the available sunlight effectively.
7. Aging and Degradation
Over time, solar cells may experience degradation, which can reduce their efficiency. Factors like exposure to ultraviolet (UV) radiation, moisture, and environmental stress can contribute to aging. Manufacturers often provide warranties that guarantee a certain level of efficiency over a specified lifespan.
Understanding these factors is crucial for optimizing solar power systems. By carefully considering and addressing these variables, it is possible to maximize the efficiency and longevity of solar cells, ensuring a reliable and sustainable source of clean energy.
Improving Solar Cell Efficiency
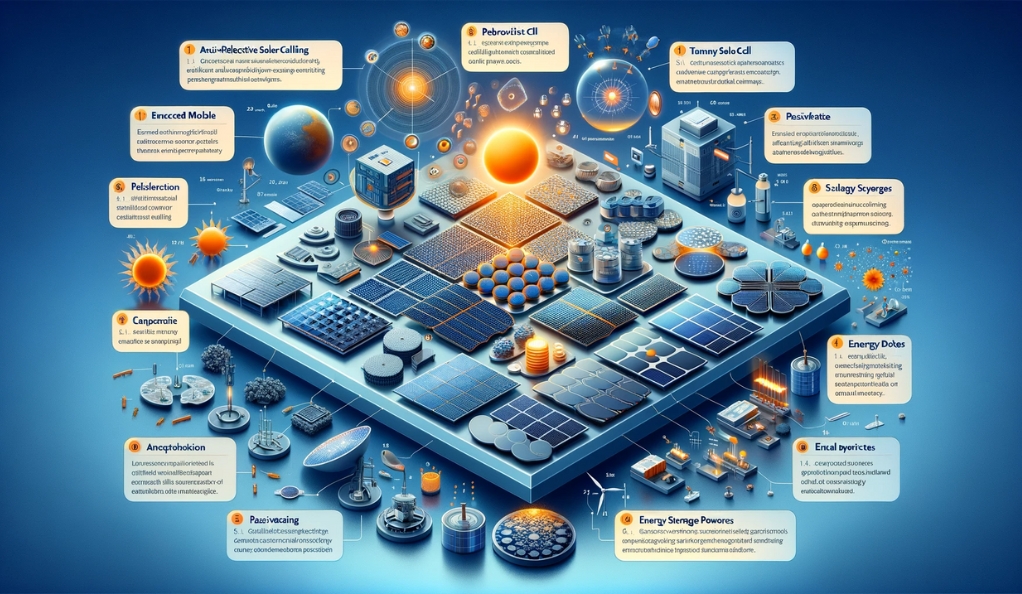
Improving solar cell efficiency is a constant pursuit in the renewable energy industry. Higher efficiency not only translates into increased electricity generation but also enhances the overall viability of solar power systems.
1. Advanced Materials
Researchers are continually developing advanced materials to improve solar cell efficiency. Some notable materials include:
- Perovskite Solar Cells: Perovskite-based solar cells have gained attention for their high efficiency and low manufacturing costs. They offer the potential to surpass traditional silicon-based cells in terms of efficiency.
- Tandem Solar Cells: Tandem solar cells stack multiple layers of different materials to capture a broader spectrum of sunlight. This approach allows for higher efficiency by optimizing absorption at various wavelengths.
2. Anti-Reflective Coatings
Anti-reflective coatings are applied to the surface of solar panels to reduce the reflection of sunlight. By minimizing reflection, more light is absorbed by the solar cells, leading to increased efficiency. These coatings are typically designed to work across a wide range of wavelengths.
3. Passivation Layers
Passivation layers are used to reduce surface recombination of electrons and holes, preventing them from recombining before contributing to electrical current. This technique enhances the overall efficiency of solar cells.
4. Bifacial Solar Panels
Bifacial solar panels are designed to capture sunlight from both the front and rear sides. This design takes advantage of reflected sunlight from the ground, increasing energy capture and overall efficiency.
5. Concentrated Solar Power
Concentrated solar power (CSP) systems use mirrors or lenses to focus sunlight onto a small area, often with highly efficient solar cells. This concentration of sunlight significantly increases the intensity and, consequently, the efficiency of electricity generation.
6. Tracking Systems
Solar tracking systems adjust the angle and orientation of solar panels to follow the sun’s path throughout the day. By maximizing direct sunlight exposure, tracking systems can significantly enhance efficiency.
7. Quantum Dots
Quantum dots are nanoscale semiconductor particles with unique properties. They can be integrated into solar cells to capture additional wavelengths of light, improving overall efficiency.
8. Improved Manufacturing Processes
Enhancements in manufacturing processes, such as improved quality control and reduced defects, contribute to higher efficiency. Precision manufacturing ensures that each solar cell performs optimally.
9. Energy Storage Integration
Efficiency can also be improved by integrating energy storage systems, such as batteries. These systems capture excess energy during periods of high generation and release it when needed, reducing energy waste.
10. Research and Development
Ongoing research and development efforts focus on exploring innovative technologies and techniques to push the boundaries of solar cell efficiency. Collaborations between academia, industry, and government agencies drive progress in this field.
By continuously improving solar cell efficiency, the renewable energy sector is making solar power more accessible, cost-effective, and environmentally friendly. These advancements pave the way for a sustainable energy future, reducing our reliance on fossil fuels and mitigating climate change.
Benefits of High-Efficiency Solar Cells
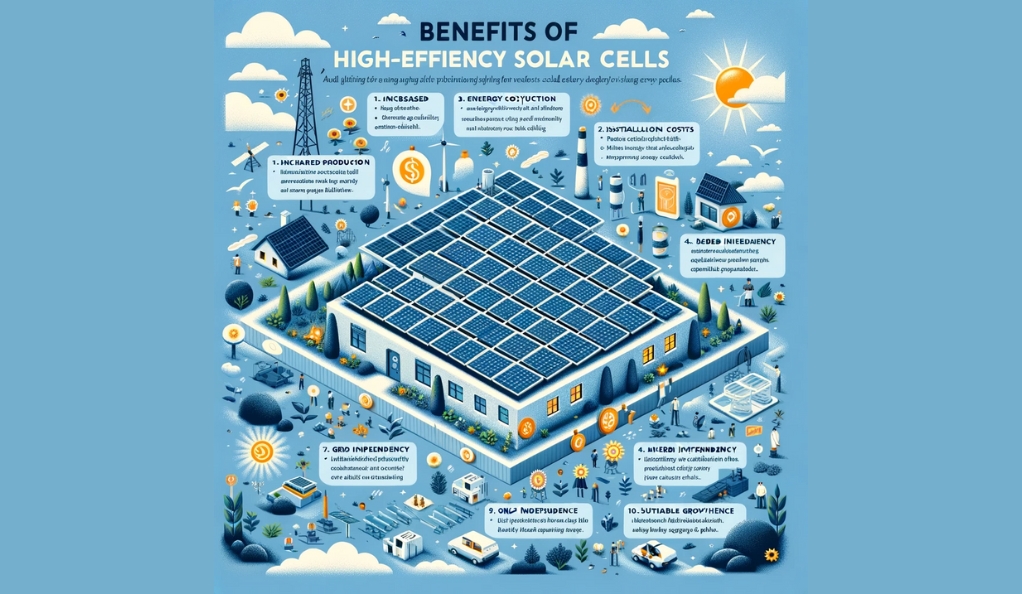
Investing in high-efficiency solar cells offers a wide range of advantages, making them a compelling choice for various applications.
1. Increased Energy Production
The primary advantage of high-efficiency solar cells is their ability to generate more electricity from the same amount of sunlight. This means that a smaller number of high-efficiency panels can produce the same power as a larger array of lower-efficiency panels. For limited rooftop or ground space, high-efficiency cells are the ideal solution, maximizing energy production.
2. Reduced Installation Costs
Since fewer high-efficiency panels are needed to achieve the desired energy output, installation costs are significantly reduced. This results in lower overall system costs, making solar power more accessible and cost-effective for homeowners and businesses alike.
3. Environmental Benefits
High-efficiency solar cells contribute to a smaller environmental footprint. They require fewer raw materials for manufacturing and less land for installation. This reduction in resource consumption and land use has a positive impact on the environment, aligning with sustainability goals.
4. Enhanced Economic Returns
Investing in high-efficiency solar panels yields higher economic returns over the lifespan of the system. With increased energy production and reduced installation costs, the payback period for solar power systems is shorter, leading to greater savings and return on investment (ROI).
5. Grid Independence
High-efficiency solar cells enable off-grid applications in remote areas. These cells can efficiently capture and store energy, providing a reliable source of electricity where traditional power sources are unavailable. This can be crucial for powering remote sensors, telecommunications equipment, and rural communities.
6. Technological Advancements
The pursuit of higher efficiency drives innovation in solar cell technology. As research and development efforts continue, advancements benefit the entire renewable energy industry. This innovation cycle leads to breakthroughs that improve efficiency and performance.
7. Reduced Maintenance
Solar panels with high efficiency typically require less maintenance. Their robust performance and reliability reduce the need for frequent cleaning and repairs, further lowering operational costs.
8. Energy Independence
High-efficiency solar cells contribute to energy independence by reducing reliance on fossil fuels and the grid. This is particularly important in regions with volatile energy markets or limited access to traditional power sources.
9. Energy Security
Solar power systems equipped with high-efficiency cells provide energy security during power outages or emergencies. Backup battery systems can store excess energy for use when needed, ensuring a continuous power supply.
10. Sustainable Growth
Investing in high-efficiency solar cells supports the growth of the renewable energy sector. It creates jobs, stimulates research and development, and fosters sustainable practices, contributing to a greener and more sustainable future.
High-efficiency solar cells offer a multitude of benefits, ranging from increased energy production and reduced installation costs to environmental sustainability and energy security. As technology continues to advance, the adoption of high-efficiency solar cells plays a crucial role in transitioning to a clean and sustainable energy landscape.
Future Trends in Solar Cell Efficiency
The field of solar cell efficiency is continually evolving, driven by innovation and the growing demand for renewable energy solutions. As we look to the future, several exciting trends and developments are poised to further enhance the efficiency and performance of solar cells.
1. Tandem Solar Cells
Tandem solar cells, which stack multiple layers of different materials, are expected to become more prevalent. These cells can capture a broader spectrum of sunlight, maximizing energy conversion efficiency. Researchers are actively exploring novel materials and designs to push the limits of tandem cell efficiency.
2. Perovskite Solar Cells
Perovskite solar cells have garnered significant attention due to their high efficiency potential and cost-effectiveness. Ongoing research aims to address stability issues and improve the durability of perovskite materials, making them a competitive choice in the solar market.
3. Bifacial Technology
Bifacial solar panels, designed to capture sunlight from both sides, are expected to see increased adoption. These panels can utilize reflected sunlight from surfaces like the ground or nearby structures, enhancing overall energy capture and efficiency.
4. Quantum Dot Technology
Quantum dots, nanoscale semiconductor particles, are being integrated into solar cells to capture a broader range of light wavelengths. This technology has the potential to improve efficiency by optimizing absorption at various parts of the solar spectrum.
5. Improved Manufacturing Processes
Manufacturers are continually refining production techniques to reduce defects, enhance material quality, and boost the overall efficiency of solar cells. Precision manufacturing ensures that each cell performs optimally, contributing to higher efficiency levels.
6. Concentrated Solar Power
Concentrated solar power (CSP) systems, which use mirrors or lenses to focus sunlight, are being deployed at larger scales. Advances in CSP technology aim to increase energy capture and overall efficiency, making these systems a more competitive alternative.
7. Artificial Intelligence (AI) Integration
AI is playing an increasingly significant role in optimizing solar energy systems. Machine learning algorithms can predict and optimize energy production, panel positioning, and maintenance schedules, ultimately improving efficiency and reducing costs.
8. Sustainable Materials
The development of sustainable and eco-friendly materials for solar cells is a growing trend. These materials not only reduce the environmental impact but also contribute to higher efficiency and performance.
9. Flexible and Lightweight Solar Panels
Advancements in thin-film and flexible solar panels are making them more versatile and suitable for a wide range of applications. These panels can be integrated into various surfaces and structures, increasing the potential for energy generation.
10. Energy Storage Integration
The integration of advanced energy storage solutions with solar power systems will continue to grow. This combination allows excess energy to be stored and used when needed, reducing energy waste and enhancing overall efficiency.
11. Space Solar Power
Innovations in space solar power systems, which capture solar energy in space and transmit it wirelessly to Earth, are being explored. These systems have the potential to provide a consistent and abundant source of clean energy.
These future trends and developments in solar cell efficiency hold the promise of revolutionizing the renewable energy landscape. As technology advances and sustainability becomes increasingly vital, solar power is poised to play a more significant role in meeting the world’s energy needs while reducing carbon emissions and environmental impact.
Conclusion
The world of solar cell efficiency is a realm of endless possibilities, where science, innovation, and environmental consciousness converge. As we’ve journeyed through the intricacies of measuring, improving, and reaping the benefits of solar cell efficiency, it is evident that the sun’s boundless energy potential holds the key to a sustainable future. From advanced materials to cutting-edge technologies, the pursuit of higher efficiency is propelling us toward a world where clean, renewable energy is not only attainable but also economically viable. With each breakthrough and emerging trend, we inch closer to a greener, more energy-independent future, where solar power plays a central role in mitigating climate change and powering our planet.
As we embrace the exciting developments in solar cell technology and efficiency, it is crucial to recognize the collective effort required to harness the sun’s energy for the betterment of humanity and the environment. The path to a sustainable energy future is illuminated by the brilliance of solar cells, and our commitment to advancing efficiency will continue to pave the way for a brighter, cleaner, and more prosperous tomorrow. So, let us harness the power of the sun, unlock its boundless potential, and usher in an era where clean, renewable energy is the driving force behind a sustainable world for generations to come.