Introduction to Quantum Dots in Solar Panels
Quantum dots (QDs), tiny semiconductor particles, have emerged as a transformative component in solar panel technology. Their ability to absorb and emit light efficiently, adjustable by their size, offers an innovative approach to enhancing solar energy conversion. Unlike traditional solar cells, quantum dots can absorb a broader range of light wavelengths, leading to higher efficiency. This tunability, combined with the potential for Multiple Exciton Generation (MEG), allows for the design of solar cells that surpass the conventional spectrum limits. While quantum dots promise significant improvements in solar panel efficiency, challenges in stability, scalability, and environmental impact remain areas of active research.
Recent advancements have led to the development of quantum dot solar cells (QDSCs) with efficiencies over 16%, showcasing the substantial potential of this technology. As researchers continue to tackle issues related to the practical implementation of quantum dots in solar panels, these nano-sized particles stand at the forefront of a shift towards more efficient and sustainable solar energy solutions. The ongoing research aims to balance efficiency, manufacturability, and environmental considerations, positioning quantum dots as a key player in the future of renewable energy.
Historical Perspective and Developmental Milestones
The development of quantum dots in solar technology began in 1989, marked by the recognition of their potential for high-efficiency solar cells. A significant breakthrough occurred in 2010 when Pattantyus-Abraham et al. demonstrated the practical use of colloidal quantum dots in photovoltaic devices, a major step in applying quantum dots in solar cell technologies. This period saw intense research into enhancing the efficiency and viability of quantum dot solar cells (QDSCs), with notable progress in understanding quantum dot surface chemistry and the exploration of hybrid quantum dot-organic solar cells.
Despite these advancements, challenges like recombination losses and stability issues persisted. However, by 2018, significant strides were made in addressing these issues. Liu’s team proposed innovative solutions to tackle recombination losses, enhancing QDSC performance. In 2020, Wang’s research focused on stability and scalability, introducing new materials and techniques to improve the practical application and manufacturing of QDSCs. These milestones underscore the evolution and potential of quantum dots in transforming solar energy efficiency.
Quantum Dot Solar Cells (QDSCs): A Technological Breakthrough
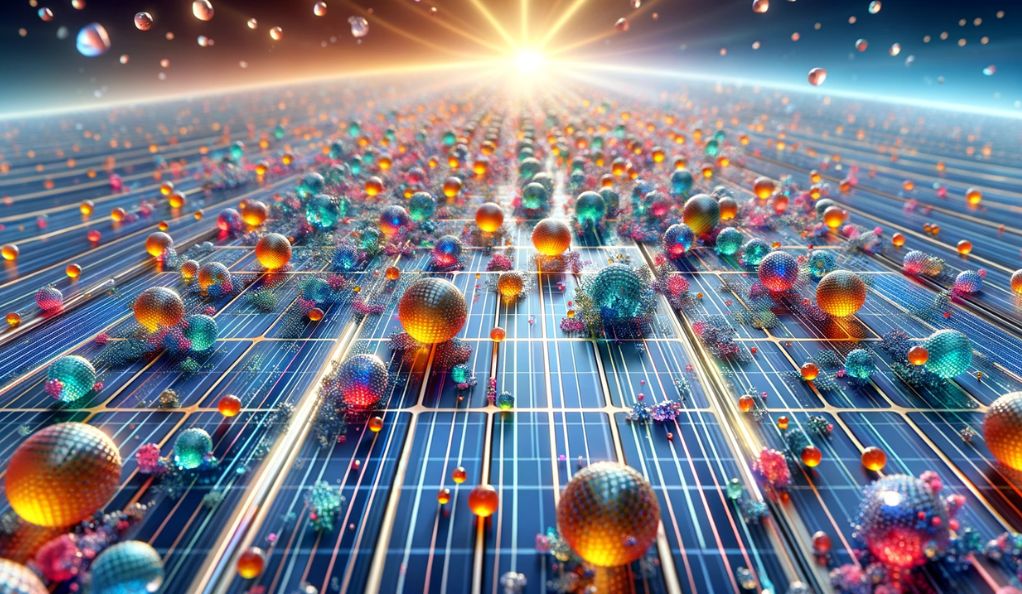
Quantum Dot Solar Cells (QDSCs) utilize quantum dots, small semiconductor particles, for enhanced solar energy conversion. These dots’ unique feature is their tunable absorption spectrum; their size determines their ability to absorb different wavelengths of light. This tunability allows QDSCs to efficiently harness a wider spectrum of solar radiation than traditional solar cells, leading to higher overall energy conversion efficiency.
The Mechanics Behind QDSC Efficiency
The efficiency of QDSCs is largely due to Multiple Exciton Generation (MEG), a process where a single photon generates multiple charge carriers, boosting the cell’s efficiency. The layered structure of QDSCs, including an electron transport layer, a quantum dot layer, and a hole transport layer, facilitates the efficient separation and collection of charge carriers generated by sunlight absorption.
Recent Advances and Milestones
Significant advancements in QDSC technology include improvements in quantum dot synthesis, leading to more uniform and effective light absorption. Integration with materials like perovskites has yielded QDSCs with efficiencies surpassing 16%. Ongoing research explores new materials and multi-junction QDSC designs to further increase efficiency, aiming to capture a broader spectrum of sunlight and push the boundaries of solar cell technology.
Enhancing Efficiency: The Science Behind Quantum Dots in Solar Cells
Quantum dots (QDs) are poised to significantly enhance the efficiency of solar cells through their unique physical properties and the innovative ways they interact with light. Central to this enhancement is their ability to absorb a wider range of the solar spectrum compared to traditional photovoltaic materials.
Tunable Band Gap and Light Absorption
The key feature of quantum dots in solar cells is their tunable band gap. The band gap of a material determines the range of light frequencies it can absorb. In quantum dots, this band gap can be precisely controlled by adjusting their size – smaller dots absorb higher-energy (blue) light, while larger ones absorb lower-energy (red) light. This tunability allows QDs to be optimized for absorbing different parts of the solar spectrum, thereby capturing more sunlight and converting it into electricity more efficiently than traditional solar cell materials.
Multiple Exciton Generation (MEG)
Another groundbreaking aspect of quantum dots in solar cells is the phenomenon of Multiple Exciton Generation (MEG). In conventional solar cells, a single photon of light typically generates a single electron-hole pair, limiting the efficiency of the cell. However, in quantum dot solar cells, high-energy photons can generate multiple electron-hole pairs. This means that quantum dots can potentially convert more of the sun’s energy into electricity, pushing the efficiency of solar cells beyond the traditional limits.
Recent Developments and Efficiencies Achieved
Recent research has focused on optimizing the integration of quantum dots in solar cells to maximize their efficiency. For instance, improvements in the synthesis of quantum dots have led to better control over their size and uniformity, directly impacting their light absorption and efficiency in solar cells. Researchers have also been exploring the combination of quantum dots with other photovoltaic materials, such as perovskites, to create hybrid solar cells with enhanced efficiency. These hybrid cells have shown promising results, with some achieving efficiencies over 16%.
Furthermore, the development of multi-junction quantum dot solar cells, where layers of quantum dots with different sizes are stacked, aims to capture a broader spectrum of sunlight, further enhancing the cell’s efficiency. These advancements are crucial in overcoming the limitations of current solar cell technologies and paving the way for the next generation of highly efficient solar energy systems.
Addressing Challenges: Stability and Scalability in QDSCs
While quantum dot solar cells (QDSCs) offer remarkable efficiency improvements, their practical application faces significant challenges, particularly in terms of stability and scalability. Addressing these challenges is crucial for the transition of QDSCs from the laboratory to widespread commercial use.
Stability Issues in Quantum Dot Solar Cells
Stability is a major concern for QDSCs. Quantum dots, especially those made from materials like cadmium selenide (CdSe) or lead sulfide (PbS), are sensitive to environmental factors such as air, light, and moisture. These factors can cause degradation of quantum dots, leading to a decline in solar cell performance over time. Research in this area has focused on developing protective coatings and encapsulation techniques to shield quantum dots from external conditions. Additionally, the exploration of new, more stable quantum dot materials is underway to enhance the longevity of QDSCs.
Scalability and Manufacturing Challenges
Scaling up the production of QDSCs to industrial levels poses another significant hurdle. The current methods of synthesizing quantum dots are complex and often involve high-cost materials and processes. Achieving a cost-effective and scalable manufacturing process is essential for the commercial viability of QDSCs. Recent advancements include the development of simpler, more efficient synthesis methods and the use of less expensive materials. These efforts aim to reduce production costs while maintaining the quality and performance of quantum dots.
Recombination Losses
Recombination losses, where electron-hole pairs recombine without contributing to electric current, are another issue that impacts the efficiency of QDSCs. Innovative approaches, such as the use of new materials for electron and hole transport layers and the improvement of quantum dot surface treatments, have been explored to minimize these losses. Research in this area focuses on optimizing the interface between quantum dots and other cell components to facilitate efficient charge separation and transport.
Research and Development Progress
The progress in addressing these challenges has been significant. For instance, the work by Liu and his team in 2018 provided insights into mitigating recombination losses. In 2020, Wang and his colleagues introduced novel materials and engineering approaches to enhance the stability and scalability of QDSCs. These advancements are critical steps towards the realization of durable, cost-effective, and high-efficiency quantum dot solar cells.
The Future of Solar Energy with Quantum Dots
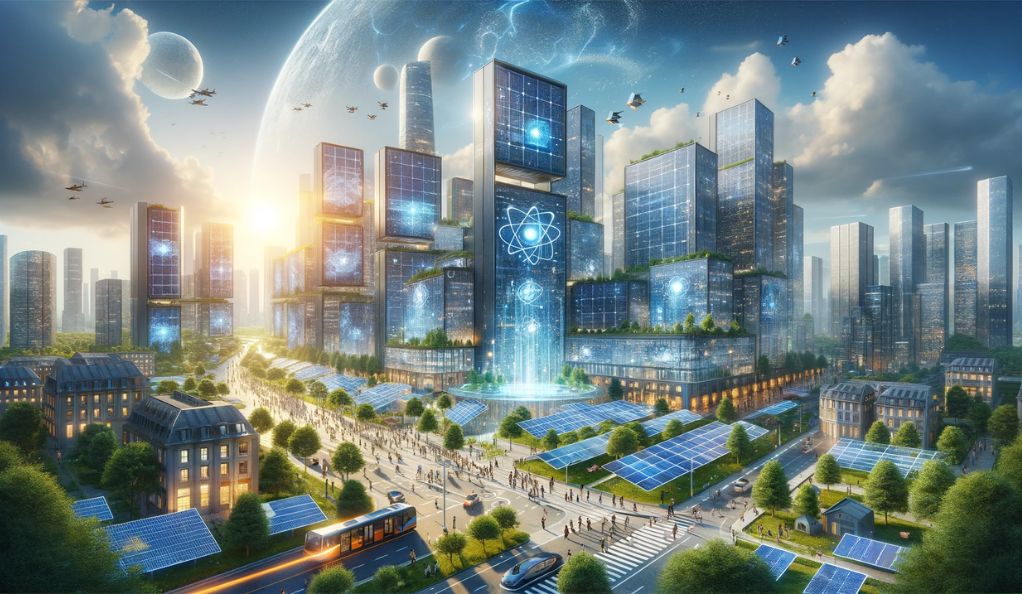
The advancements in Quantum Dot Solar Cells (QDSCs) not only represent a remarkable scientific achievement but also herald a new era in solar energy technology. The future of solar energy, with quantum dots at its core, is poised to be more efficient, cost-effective, and environmentally sustainable.
Potential for Higher Efficiency
One of the most exciting prospects for QDSCs lies in their potential to significantly exceed the efficiency limits of current solar panel technologies. Researchers are working towards achieving efficiencies as high as 30% in the near future. This is a substantial leap from the current efficiencies of traditional solar panels, which average around 15-20%. The ability of quantum dots to absorb a broader spectrum of sunlight and convert it into electricity is key to this enhanced performance.
Integration with Existing Solar Technologies
Quantum dots can be integrated with existing solar technologies, such as perovskite solar cells, to create hybrid systems that combine the best attributes of both technologies. These hybrid systems can potentially offer higher efficiencies, better stability, and lower manufacturing costs. Such integrations not only improve performance but also pave the way for more versatile solar energy solutions, adaptable to various applications and environments.
Environmental Impact and Sustainability
The environmental impact of solar technology is a critical consideration. Quantum dots, particularly those made from less toxic materials, can offer a more environmentally friendly alternative to some traditional photovoltaic materials. Research in this area is focused on developing quantum dots using sustainable materials and processes, reducing the overall ecological footprint of solar cell production.
Market Potential and Commercialization
The commercialization of QDSCs is another important aspect of their future. As research continues to overcome the challenges of stability and scalability, quantum dots are becoming more viable for mass production. The market potential for high-efficiency, cost-effective solar panels is enormous, and quantum dots could play a central role in meeting the growing global demand for renewable energy sources.
Ongoing Research and Collaborations
Collaborations between academic institutions, research laboratories, and industry are crucial in driving the advancements in QDSC technology. These partnerships facilitate the sharing of knowledge, resources, and expertise, accelerating the pace of innovation and bringing quantum dot solar cells closer to widespread practical application.
Quantum Dots and Environmental Sustainability
The sustainability of quantum dot solar cells (QDSCs) is a crucial aspect of their development, focusing on eco-friendly materials and efficient manufacturing processes. The shift from traditional heavy metal-based quantum dots to less toxic materials is a key research area, aimed at reducing environmental risks while maintaining efficiency. The entire lifecycle of QDSCs, including production, usage, and disposal, is under scrutiny to ensure minimal environmental impact. This approach includes exploring energy-efficient manufacturing methods and effective recycling strategies for end-of-life panels.
Regulatory frameworks and policy support are also essential to promote sustainable practices and encourage the use of environmentally friendly materials in the solar industry. As QDSC technology advances, addressing these sustainability factors is imperative to ensure that they not only provide a high-efficiency renewable energy solution but also align with global environmental and sustainability goals.
Conclusion: Quantum Dots and the Horizon of Renewable Energy
In conclusion, quantum dot solar cells (QDSCs) represent a significant breakthrough in solar energy technology, offering a promising future for renewable energy. From their early conceptualization to recent advancements, QDSCs have consistently demonstrated the potential to exceed traditional solar cell efficiencies. Challenges like stability, scalability, and environmental sustainability are being actively addressed, paving the way for their practical application. The integration of quantum dots with existing solar technologies, coupled with ongoing research and development, is steering us towards a future where solar energy is not only more efficient but also more accessible and environmentally sustainable. The journey of QDSCs, marked by continuous innovation and improvement, highlights the transformative potential of quantum dots in reshaping the solar energy landscape.